Tuned Oscillators Pdf
Replacing YIG-Tuned Oscillators with Silicon by Using an Ultrawideband PLL/VCO with Precision Phase ControlRF and microwave instruments, such as signal and network analyzers, require wideband, swept frequency signals to make many of their basic measurements. Yet, perversely, wideband voltage controlled oscillators (VCOs) typically have the worst phase noise due to the low Q and high K VCO (a VCO’s tuning sensitivity in MHz/V) required to maximize their tuning ranges. Yttrium iron garnet (YIG) tuned oscillators (YTOs) neatly solve this problem with good, wideband phase noise performance and an octave of frequency tuning range but can be large, expensive, and can consume hundreds of mA current due to their tuning currents. And of course they still require an external phase-locked loop (PLL) to close the loop and a voltage controlled current source to provide their tuning current.A YIG crystal sphere looks like a high Q LC circuit whose resonant frequency is linearly proportional to an externally applied magnetic field. The oscillator is tuned by a current through a single turn loop through an octave or more in the GHz range.
YIG tuned oscillators exhibit a low level of phase jitter, and their broadband characteristics from approximately 2 GHz to 18 GHz (with a very linear tuning curve) make them a popular choice for many measurement applications.However, the performance gap between YIG-tuned oscillators and integrated PLL/VCO ICs is closing. For example, recent advances in integrated PLL/VCO ICs, such as the Analog Devices, have much improved phase noise over their predecessors. They have also solved the wide tuning range issue by design techniques such as dividing the output frequency range into multiple, adjacent subbands, where each subband can have a dedicated band switched VCO to increase tuning range while making the band switched VCOs look like a single VCO with a moderate K VCO (shown in Figure 1) to the user. Next, auxiliary frequency dividers and doublers extend the frequency coverage of the VCO’s tuning range both above by multiplication and below by division. For example, the ADF4355’s fundamental tuning range of 3.4 GHz to 7.2 GHz is extended down to 54 MHz by division.
Each time the frequency is halved the phase noise improves by 3 dB (shown in Figure 2). Each time the output frequency is halved, the overall phase noise improves by 3 dB. In this case, dividing the 3.4 GHz VCO by 64 GHz results in phase noise of better than −130 dBc/Hz at a 10 kHz offset at 53.125 MHz.Yet, even if an integrated PLL/VCO IC has a more comparable tuning range than a YIG-tuned oscillator, one problem still remains: YIG-tuned oscillators still provide 12 dB better phase noise performance than the best integrated VCOs.
Even this gap can be reduced if not yet closed, by combining the outputs of multiple PLL/VCOs in parallel (as shown in Figure 3). The outputs can be summed and the phase noise improved by 3 dB for each doubling (3 dB) of the number of PLL/VCOs in parallel. For example, two ADF4355 PLL/VCOs provide a 3 dB improvement, four ADF4355 PLL/VCOs provide a 6 dB improvement, and eight ADF4355 PLL/VCOs provide a 9 dB improvement (as shown in Figure 4). By phase locking and combining the outputs of eight ADF4355 PLL/VCOs you can improve the overall phase noise by nearly 9 dB over that of a single PLL/VCO. The spectrum here shows both the output phase noise of a single ADF4355 and the phase noise of the summed outputs of eight synchronized ADF4355s operating in parallel.The key to summing the outputs of the PLL/VCOs is operating the outputs of all the oscillators in phase.
The example we’ll discuss here uses four PLL/VCOs in parallel. As one might guess, placing four phase-locked loops and voltage controlled oscillators at the same frequency, on the same printed circuit board, carries with it a number of challenges.
Chief among these challenges is isolation. Poor isolation between PLLs can result in a phenomenon known as injection locking (as seen in Figure 5), in which an oscillator locks to a strong signal or harmonic thereof, in preference to the frequency selected by the tuning voltage supplied from the phase-locked loop itself. Injection locking is observable by seeing an initially subtle degradation in noise and spurious, as the two locking mechanisms generate intermodulation distortion. In more severe cases the signal will look more like a modulated carrier than a continuous sine wave tone. Injection locking occurs when the frequency of a VCO locks to an external oscillation rather than its control voltage. The result is increased intermodulation and phase noise.Isolation requires a variety of techniques and circuitry. For example, buffer the reference signal to each PLL (pins REF INA and REF INB) by using a buffer, in this case the ADI LVPECL 8:1 clock buffer.
Additionally, minimizing crosstalk requires correct terminations of the source, and load pins as close to the source and load as possible. Extra shunt capacitance to ground (18 pF) was also included to attenuate any leakage from the VCO’s output while passing the desired reference frequency.Other paths requiring isolation are the power supply lines. To provide the required isolation, each PLL has its own high performance regulator (the ADI ), one for the +5 V lines, V VCO, VP, and V REGVCO, the VCO supplies being the more critical here. The analog (AV DD), digital (DV DD), and output stage (V RF) lines also require 3.3 V, and separate regulators for each line are also used. It is acceptable to tie the 3.3 V lines on each PLL together provided that good decoupling has been used.On the RF output stage, the auxiliary output (pins RF OUTB+ and REF OUTB–) is disabled and terminated to ensure no unwanted tones are generated. Output RF OUTA– is terminated in a 50 Ω load, and its complementary output, RF OUTA+, is fed to a high isolation power combiner (Marki Microwave, PBR0006SMG).
This combiner was chosen to ensure that coupling between the output stages is kept to a minimum while providing a combined signal at the common output. To improve isolation, a pair of these combines the outputs of two PLLs, and a third combiner sums the output of the first two.Finally, an off the shelf shield from Laird provides additional isolation to minimize any stray radiation that might couple the VCOs electromagnetically. Taken together, all of these steps ensure the best possible isolation.The ADF4355 contains a high resolution 24-bit modulator, which in addition to allowing the generation of fractional-N divide values, also contains circuitry, which permits small adjustments of the phase of the RF signal.
To be useful, the phase values need to be repeatable. This requires the use of the function phase resync.Phase resync is best described as a function that puts the fractional divider (a ∑-∆ modulator with noise shaping) into a known state after a frequency update. Because phase is a relative measurement, the resync function is defined as functional when changing from a frequency F1 with phase P1 to frequency F2, and when changing back to frequency F1, the phase should again be P1 as in the first measurement.
This function combined with resync allows us to adjust the phase to minimize the phase difference between each of the four PLLs to get the maximum power sum of the power from the four PLLs and thus, the biggest improvement in phase noise. In addition to these steps, it is also important to reset the counters of each PLL simultaneously, and this is easily achieved by a hardware power-down and power-up using the chip enable (CE) pin.Process and part to part variation means that we cannot assume that the phase difference between each PLL, when following reset and resync procedures, will be close enough to zero to maximize signal-to-noise; thus an external calibration circuit is required.The calibration procedure is simple: turn on a single PLL/VCO and define its phase as phase zero.
Turn on each additional PLL/VCO in turn, change its output phase until the combined output power of the on PLL/VCOs is at a maximum, then turn on the next VCO and again tune its phase until the combined output power of the PLL/VCOs is again at a maximum. Note, that the largest change in power occurs when the second PLL/VCO is turned on as the combined power is doubled; the difference decreases for each subsequent PLL/VCO. In practice this means the signal-to-noise increases each time the number of PLL/VCOs in parallel doubles. That is, two in parallel provide a 3 dB improvement, four provide a 6 dB improvement, and eight provide a 9 dB improvement. Of course the complexity of the power combiner doubles as well, so four PLL/VCOs is a practical upper limit with diminishing returns for eight and 16 PLL/VCOs in parallel.Note, that best phase performance and maximum output power are coincidental, so a power measurement is sufficient to ensure best phase noise performance.
The calibrator in our case is an ADI power detector to measure the output amplitude of the combined signals. In this manner the phase of each PLL (at each frequency) can be adjusted, and the phase adjustment constant when the combined power has reached a maximum (as shown in Figure 6). This process is repeated for each additional PLL until all four PLLs have been powered up and adjusted, such that the signal at the output of the combiners is at a maximum. Four phase aligned ADF4355 integrated PLL/VCOs shown with an ADCLK948 clock buffer, combiners (PBR-0006SMG), and calibration circuitry.Figure 7 shows that practical results follow theory, that for each doubling of PLL/VCOs and with the correct phase word written, the combined phase noise of the four PLL/VCOs improves by 6 dB over that of a single PLL/VCO. Thus, the performance of one ADF4355 PLL (–134 dBc/Hz at a 1 MHz offset) can be improved by 6 dB to about –140 dBc/Hz when four PLL/VCOs are combined in phase.
Ian CollinsIan Collins graduated from University College Cork with a degree in electrical and electronic engineering and has worked in the RF and Microwave Group of Analog Devices since 2000. He is currently applications manager of the Microwave Frequency Generation Group, which focuses mainly on phase-locked loop (PLL) and voltage controlled oscillator (VCO) products. When not spending time at work or with his young family, Ian enjoys photography and the theater (both on- and off-stage), reading, and listening to music.Related Products.Two Selectable Inputs, 8 LVPECL Outputs SiGe Clock Fanout Buffer.Microwave Wideband Synthesizer with Integrated VCO.Fast Responding, 45 dB Range, 0.5 GHz to 43.5 GHz Envelope Detector.800 mA, Ultra Low Noise/High PSRR LDORelated Markets & Technology.
The cookies we use can be categorized as follows: Strictly Necessary Cookies: These are cookies that are required for the operation of analog.com or specific functionality offered. They either serve the sole purpose of carrying out network transmissions or are strictly necessary to provide an online service explicitly requested by you. Analytics/Performance Cookies: These cookies allow us to carry out web analytics or other forms of audience measuring such as recognizing and counting the number of visitors and seeing how visitors move around our website. This helps us to improve the way the website works, for example, by ensuring that users are easily finding what they are looking for. Functionality Cookies: These cookies are used to recognize you when you return to our website. This enables us to personalize our content for you, greet you by name and remember your preferences (for example, your choice of language or region). Loss of the information in these cookies may make our services less functional, but would not prevent the website from working.
Targeting/Profiling Cookies: These cookies record your visit to our website and/or your use of the services, the pages you have visited and the links you have followed. We will use this information to make the website and the advertising displayed on it more relevant to your interests. We may also share this information with third parties for this purpose.
IndroductionAn LC oscillator is actually a which uses capacitors and inductors in its feedback network. It can be built from a transistor, an operational amplifier, a tube, or some other active (amplifying) device. Oscillation is brought about by applying a portion of the amplifiers’ output signal to its input.
That feedback signal must be applied in phase with the original input signal. The amplifier is usually an inverter that provides 180 o of phase shift by itself, and an additional 180 o of phase shift must be provided through some other means.In an LC oscillator circuit, the feedback network is a tuned circuit (often called a tank circuit). The tuned circuit is a resonator consisting of an inductor (L) and a capacitor (C) connected together. Charge flows back and forth between the capacitor's plates through the inductor, so the tuned circuit can store electrical energy oscillating at its resonant frequency. There are small losses in the tank circuit, but the amplifier compensates for those losses and supplies the power for the output signal. LC oscillators are often used at radio frequencies, when a tunable frequency source is necessary, such as in signal generators, tunable radio transmitters and the local oscillators in radio receivers. Typical LC oscillator circuits are the Hartley, Colpitts and Clapp circuits.TopologyIn an LC oscillator, the feedback network is mainly composed of three impedances (see figure 1).
All three impedances Z1, Z2 and Z3 are pure reactance elements, implying that they can be either capacitive or inductive, depending on our design needs. In figure 1, for convenience, we have shown the amplifying element as an operational amp, but other devices could be also used. LC oscillator topologyBy looking at figure 1, you’ll see that the feedback factor (the voltage gain of the feedback network), B(f), can be stated in a form that resembles the equation describing a resistive. (3)Where A(f), B(f) are the amplifier’s gain and the feedback gain, respectively. In general, both the amplifier and the feedback network will alter the magnitude and the phase of the signal. So, both A and B are complex values, and each value has magnitude and argument (phase).The Colpitts oscillatorA feedback oscillator which uses capacitors C1 and C2, for Z1 and Z2, and an inductor L1, for Z3, is known as a “Colpitts” oscillator (see figure 2). The Colpitts oscillator was invented in 1918 by the American engineer.
Tuned Oscillators Pdf Free
The Colpitts circuit, like other LC oscillators, consists of a gain device (such as a bipolar junction transistor, field effect transistor, operational amplifier, or vacuum tube) with its output connected to its input in a feedback loop containing a parallel LC circuit (tuned circuit) which functions as a bandpass filter to set the frequency of oscillation. Colpitts oscillator topologyThe frequency of oscillation is actually the frequency. At resonance, total capacitive and total inductive reactances, are canceled out. (7)Equation (7) states that, at resonance, the feedback factor is a purely negative number, which means that the feedback network provides 180 o (π rad) of phase shift by itself.
By using an inverting amplifier (which also provides 180 o of phase shift), there is no doubt that the condition stated by equation (3), is well satisfied.Now, let’s examine if the condition stated by (2) is also satisfied:In our example, the amplifier is an inverting amplifier, based on an op-amp. So, its voltage gain is given. (9)Equation (9), states that the circuit will oscillate, if the gain of the amplifier is equal or greater than C1/C2.
So C1/C2 is the minimum gain required. In practice, the C1/C2 ratio is a trade-off between amplitude and stability. By choosing the minimum gain, the total loop gain will be equal to 1 and the amplitude of the oscillations will remain constant. However, there are inevitable tolerances. So, in practice, we must choose a much greater gain, to ensure that oscillations will always start-up.
At a much greater gain, the amplitude of the oscillations will rapidly grow up with time, until the amplifier will be saturated and it will start clipping.The Hartley oscillatorThe Hartley oscillator was invented in 1915 by American engineer. The distinguishing feature of the Hartley oscillator is that the tuned circuit consists of a single capacitor in parallel with two inductors in series (or a single tapped inductor), and the feedback signal needed for oscillation is taken from the center connection of the two inductors. Hartley oscillator topologyThe classic Hartley oscillator is shown in Figure 3. The principal difference between the Hartley and the Colpitts oscillators is the type of reactance elements used for Z1, Z2, and Z3. In the Colpitts oscillator, Z1 and Z2 are capacitive, but in the Hartley version they’re inductive. A Hartley oscillator may use a tapped inductor, but is still a Hartley oscillator.
Further, Z3 is inductive in the Colpitts, but capacitive in the Hartley. The circuit of figure 3 will oscillate (see equation 4) at a frequency, f o, given.
(10)Like the Colpitts oscillator, the nominal gain of the Hartley oscillator is R2/R1, and the minimum gain is L1/L2.The Clapp oscillatorThe Clapp oscillator is actually a modified version of the Colpitts oscillator. The Clapp oscillator is a Colpitts oscillator that has an additional capacitor placed in series with the inductor.
It was published by in 1948. Clapp oscillator topologyReferring to the notional circuit in figure 4, the network comprises a single inductor and three capacitors. Capacitors C1 and C2 form a voltage divider that determines the amount of feedback voltage applied to the amplifier imput. The oscillation frequency can be found from (4), and it is. (11)A Clapp circuit is often preferred over a Colpitts circuit for constructing a variable frequency oscillator (VFO). In a Colpitts VFO, the voltage divider contains the variable capacitor (either C1 or C2). This causes the feedback voltage to be variable as well, sometimes making the Colpitts circuit less likely to achieve oscillation over a portion of the desired frequency range.
This problem is avoided in the Clapp circuit by using fixed capacitors in the voltage divider and a variable capacitor (C 0) in series with the inductor.Like the Colpits oscillator, the nominal gain of the Clapp oscillator is R2/R1. And the minimum gain is C1/C2.Practical circuitsA discrete-transistor implementation of the Colpitts oscillator is shown in Figure 5a. The split-capacitor voltage divider that provides feedback consists of C2 and C3. In general, C3 has a larger value than C2. Resistor R3 biases the transistor, and R1 provides stability. The output signal is developed across R1. The frequency of oscillation is determined by the resonant tank circuit Z, which is connected to the base of the transitor via C4 as shown.The tank circuit is composed of a coil and a capacitor, which may be connected in series, as shown in Figure 5b, or in parallel as shown in Figure 5c.
The series circuit is a Clapp oscillator. Figure 5. The practical Colpitts oscillator uses the parallel tank circuit. The series circuit is a Clapp oscillatorA Hartley oscillator may be built as shown in Figure 6.
Again, a bipolar transistor is the gain-producing element. Resistors R1 and R2 bias Q1, and serve no other purpose. The oscillating frequency is determined by the resonant tank circuit that is made up of L1 and C1, and the output signal can be taken either from the emitter of the transitor via C5, or from coil L2, which is coupled to L1. The position of L1’s tap is a trade-off between the stability and the amplitude of the output signal. A typical Hartley oscillatorBoth Hartley and Colpitts oscillators are common-collector circuits, because the collector of the transistor is at AC ground by virtue of the presence of a bypass capacitor – that capacitor is C5 in figure 5a, and C4 in Fihure 6.The Armstrong oscillatorThere are many additional types of LC oscillators, but two are particularly interesting, historically speaking. The Armstrong oscillator is named after who invented the regenerative detector, the super-heterodyne radio, frequency modulation, and other things.
A practical circuit of an Armstrong oscillator is shown on figure 7. That circuit uses a FET as the amplifying element; the original Armstrong circuit used a tube. Another popular name of the Armstrong oscillator is the “tickler oscillator”, we’ll see why in a moment.
Sine Wave Oscillators Pdf
A typical Armstrong oscillatorThe Armstrong circuit’s frequency of oscillation is determined by the values of the components in the parallel-resonant tank circuit composed of C1 and L1. A feedback coil or “tickler” coil, L2, is closely coupled with L1, and the tickler serves to feed part of the output signal back to the input. Care must be taken to be sure that the mutual inductance between L2 and L1 is of the proper “polarity”. Otherwise, feedback will occur with the wrong pahse, and the circuit won’t oscillate.The amount of feedback, or regeneration, is controlled by a variable resistor R3; that resistor controls the amount of current flowing in the tickler coil. In older circuits, the tickler coil was wound on a mechanism that permitted the position of the coil to be varied with respect to L1.When coupling was maximum, so was feedback. The frequency of oscillation of the Armstrong oscillator may be calculated by plugging the values of L1 and C1 into the resonance equation (4), that we indroduced earlier in this article.The TITO oscillatorOur final LC oscillator has been called by several different names in the past. In older texts that illustrate the use of vacuum-tube circuits, the circuit is called a Tuned-Grid-Tuned-plate (TGTP) oscillator.
More recently it has been called a Tuned-Base-Tuned-Collector (TBTC) oscillator. Perhaps now that we have so many types of amplifying devices, the circuit should be called a Tuned-Input-Tuned-output (TITO) oscillator.Whatever we call it, it looks something like the circuit shown in figure 8.The frequency of oscillation of the circuit is set by both the L1/C1 and L2/C2 tank circuits. Most textbooks recommend that the two tank circuits resonate at almost equal but slightly different frequencies. The TITO oscillatorFeedback in the TITO oscillator is brought about through inter-element capacitance in the transistor. In other words, Cbe (the capacitance between base and the emitter) and Ccb (the capacitance between collector and base) are not external capacitors; they are part of the structure of the transistor.
FCE Gold Plus corresponds to level B2 of the Common European Framework.The Maximiser is a unique combination of workbook and exam handbook, which can be used for immediate follow-up work in class or for homework, intensive exam preparation after completing the Coursebook, or by students preparing for the exam independently. 9Gold Plus is the updated edition of Gold, the trusted exam preparation course for adult and young adult learners. Fce gold plus 2008 coursebook pdf answers.
That is why they have been shown in dashed lined in the illustration.The TITO oscillator is rarely used these days. That sort of circuit may appear to have no capacitors and only inductors but don’t be fooled. The designers of the circuit indented for stray device and circuit capacitance to be sufficient to produce oscillation at the desired frequency.ConclusionWe’ve covered quite bit of basics in this article. We have covered the theoretical background of LC oscillators.
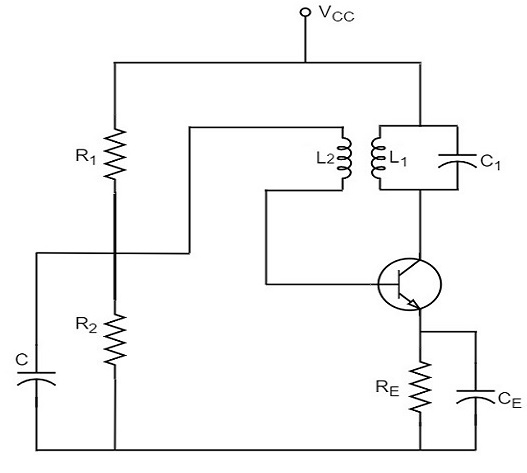
Actually, the theoretical background of LC oscillators is based on the general concepts of the. Then, we went to look at Colpitts, Hartley, and Clapp oscillators. After examining them theoretically, we discussed several practical circuit implementations.
Finally we discussed several historically interesting circuits: The Armstrong oscillator and the Tuned-Input-Tuned-output (TITO) oscillator. When we continue in a future article, we’ll discuss RC oscillators that may be built from op-amps.